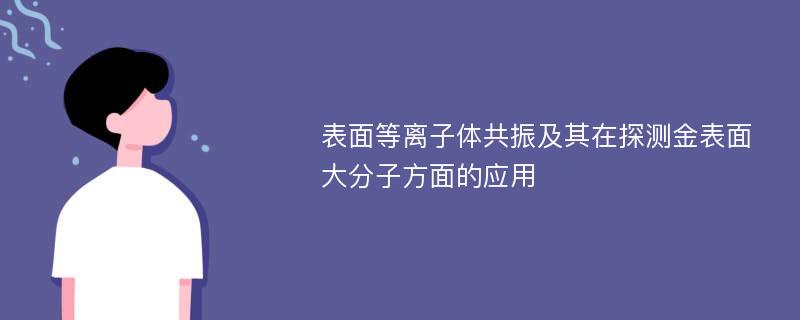
论文摘要
表面等离子体共振(surface plasmon resonance)可以存在于金属和电介质的交界面上,它的局域电场放大效应使得它对表面的特性非常敏感。在本论文中表面等离子体共振技术被用来研究金表面的大分子自组装效应。我们首先进行了一些实验和理论的工作来提高表面等离子体共振探测器的性能。通过对表面等离子体共振系统用多层膜模型来模拟,并且用菲涅耳理论来处理它的反射,我们研究了实验结果和理论模拟之间差别的来源。我们发现这种差别主要并不是由金表面的粗糙引起的,而是由金膜的紧密度引起的。我们改进了金膜的制备方法来减小实验结果与理论计算之间的差别。通过高沉积速度制备的金膜具有更高的紧密性,并且它的表面更平整,因此更适合制备高性能的SPR探测器。自组装单层膜(self-assembed monolayers)被制备在金表面上用来帮助吸附DNA等大分子。我们成功的制备了几种不同的自组装单层膜,并且通过电化学阻抗谱(electrochemical impedance spectroscopy)测试了它们的特性。此外,我们还通过表面等离子体共振技术实时监测了自组装单层膜形成的动力学过程。我们证明了通过巯丙基三甲氧基硅烷((3-Mercaptopropyl)trimethoxysilane)和3-氨丙基三乙氧基硅烷(3-Aminopropyl-triethoxysilan)构造的双层自组装单层膜来吸附DNA分子是可行的,但是它的效率不高。我们通过电化学阻抗谱和表面等离子体共振详细研究了巯基十一烷醇(11-Mercaptoundecanol)和11-氨基-1-十一烷硫醇(11-Amino-1-undecanethiol)分子构成的混合自组装单层膜。因为在缓冲液中巯基十一烷醇分子是不带电的,但是11-氨基-1-十一烷硫醇分子可以带有正电荷,具有自组装单层膜的表面的电荷可以通过改变这两种分子的比例以及缓冲液的特性来调节。我们研究了他们与溶液离子力以及酸碱度的关系。这种巯基十一烷醇/11-氨基-1-十一烷硫醇的自组装单层膜具有很好的电流阻断特性。此外,我们还研究了它们对施加电压的反应,这些自组装单层膜在一个很小的电压范围内丧失这种好的电流阻断特性,这个范围相对于银/氯化银标准参考电极一般为[-100mV,0mV]。为了制备基于表面等离子体共振的DNA芯片,我们致力于研究DNA在这些巯基十一烷醇/11-氨基-1-十一烷硫醇的混合自组装单层膜表面的吸附。我们通过表面等离子体共振测量了DNA吸附在这些混合自组装单层膜上的动力学过程。研究的DNA有两种,一种为3000对基的环形质粒DNA,另外一种为200对基的直DNA。我们分别研究了DNA吸附对于溶液中DNA的浓度、表面电荷、溶液离子力的关系。吸附在金表面的DNA的拓扑图也用原子力显微镜进行了观察。通过这两种方法的结合,我们可以提取DNA吸附的很多信息。为了从DNA芯片上提取基因信息,我们提出利用表面等离子体共振的电场增强效应构建一种新的灵敏的表面二次谐波显微镜。数值模拟的结果表明这种表面等离子体共振增强二次谐波显微镜能够探测更多的表面二阶非线性极化系数。它还具有高分辨率表面成像和探测表面分子取向的潜力。
论文目录
摘要AbstractRésuméAcknowledgementsPreamblePreparation of high performance gold layers and their characterizationPreparation of SAMs and their characterizationAdsorption of DNAs on SAMs and their characterizationDeveloping a SPR enhanced second harmonic generation microscopyConclusion1 Introduction to Surface Plasmon Resonance1.1 Fundamentals of SPs1.1.1 Definitions of SPs1.1.2 History and applications of SP techniques1.1.2.1 Ultra-sensitive detectors1.1.2.2 Plasmonics1.1.3 Dispersion relation of SPR1.1.4 Optical coupling methods1.1.5 Various optical configurations to excite SPR1.2 Description of our SPR experiment1.2.1 Optical systems to generate SPR1.2.2 Micro-fluid systems1.2.3 Preparation of metal films1.3 Computation of reflected and transmitted fields1.3.1 Reflection and transmission of optical waves in isotropic layered media1.3.1.1 Snell's law1.3.1.2 Fresnel's formulae1.3.1.3 Total internal reflection and critical angle1.3.1.4 Evanescent wave and attenuated total reflection1.3.2 Matrix formulation for isotropic layered media1.3.2.1 Transmission and propagation matrices1.3.2.2 Matrix formulation for a multilayer system1.3.2.3 Application of matrix formulation on the modeling of SPR1.3.2.4 The distribution of the transmitted and reflected fields1.3.2.5 Influence of variation of refractive indices on SPR effect1.3.2.6 Influence of excitation wavelength on SPR effect1.3.2.7 Influence of the thickness of gold layer on SPR effect1.4 Fine size effects1.4.1 Focalization of the beam1.4.2 Parallelism of the exciting beam1.5 Conclusion2 Effect of inhomogeneity of gold on SPR reflectivity curves2.1 Methods of gold thermal vaporization2.1.1 Slow thermal deposition2.1.2 Fast thermal deposition2.2 Set(A)of slow and fast gold vaporization2.2.1 Experimental configuration for polarization2.2.2 Slow gold vaporization2.2.2.1 AFM characterization2.2.2.2 SPR characterization2.2.3 Reproducibility of the slow deposition2.2.3.1 AFM characterization2.2.3.2 SPR characterization2.2.4 Fast gold vaporization2.2.4.1 AFM characterization2.2.4.2 SPR characterization2.2.5 Aging of the gold layers2.2.5.1 AFM characterization2.2.5.2 SPR characterization2.3 Set(B)of slow and fast gold vaporization2.3.1 Experimental configuration for polarization2.3.2 Slow gold vaporization2.3.2.1 AFM characterization2.3.2.2 SPR characterization2.3.3 Fast gold vaporization2.3.3.1 AFM characterization2.3.3.2 SPR characterization2.3.4 Reproducibility of the series(B)2.3.5 Preliminary conclusions2.4 Effective medium model for gold vaporized layers2.4.1 Maxwell-Garnet and Bruggeman model2.4.2 Roughness of gold surfaces modelling with an effective layer2.4.2.1 Slow deposition mode2.4.2.2 Fast deposition mode2.4.3 Fitting slow and fast gold deposition with modified gold index values2.4.3.1 Slow deposition mode2.4.3.2 Fast deposition mode2.4.4 Scanning Surface Plasmon Microscopy Images2.4.5 AFM images of fast and "chopped" deposition gold surfaces2.5 Conclusion3 Self-Assembed Monolayers3.1 Introduction to SAMs3.1.1 Methods of preparing ultrathin layers3.1.1.1 Spin coating3.1.1.2 Langmuir-Blodgett technique3.1.1.3 Self assembled monolayer(SAM)3.1.2 SAMs of alkanethiol molecules on Au3.1.2.1 The reason of choosing on gold to prepare SAMs3.1.2.2 Method of preparing SAMs3.1.2.3 Structure of alkanethiol SAMs on Au3.1.2.4 Other results3.1.3 Methods of characterization of SAMs3.1.3.1 AFM technique3.1.3.2 EIS technique3.2 Preparation of SAMs3.2.1 Preparation of double SAMs of MPTS and APTES3.2.2 Preparation of mixed SAMs of MUO and AUT in ethanol3.2.3 Preparation of mixed SAMs of MUO and AUT in buffers3.3 Results3.3.1 AFM images of SAM surface3.3.1.1 AFM images of Double SAMs of MPTS and APTES3.3.1.2 AFM images of of mixed SAMs of MUO and AUT3.3.2 Formation of SAMs studied by SPR3.3.2.1 Description of experiments3.3.2.2 Experimental results3.3.3 Characterization of SAMs by EIS3.3.3.1 Description of EIS experiments3.3.3.2 Modelling the interracial impedance3.3.3.3 Typical curves recorded during an EIS experiments3.3.3.4 Modulation of the gold potential during an EIS experiment3.3.3.5 Potential response of an uncharged MUO SAM3.3.3.6 Potential response of a charged AUT SAM3.3.3.7 Potential response of mixed SAMs3.3.3.8 Conclusion of the EIS study of SAMs on gold4 Characterization of DNA adsorption by SPR4.1 The purpose of studying the adsorption of DNA4.1.1 Introduction to the structure of DNA4.1.1.1 Chemical composites of DNA4.1.1.2 Physical structures of DNA4.1.2 DNA chips4.1.3 Dynamics of DNA4.1.3.1 Spontaneous bending of DNA4.1.3.2 Long range correlation in DNA4.2 Adsorption of long chain DNA4.2.1 Influence of DNA concentration on DNA adsorption4.2.2 Influence of surface charges on DNA adsorption4.2.3 Influence of the solution ionic strength on DNA adsorption4.3 Adsorption of short DNA chain4.3.1 Influence of MUO/AUT proportion on DNA adsorption4.3.2 Comparison of the adsorption of long and short DNAs5 SPR enhanced surface second harmonic generation5.1 Introduction to SSHG5.2 SPR enhanced SSHG5.2.1 Dependence of field enhancement on gold layer thickness5.2.2 Description of experiment and primary results5.3 SPR enhanced SHG microscopy5.3.1 Excitation of SPR enhanced SSHG by objective lens5.3.2 Electromagnetic field near focus5.3.2.1 Scalar diffraction theory5.3.2.2 Vectorial field distribution in a stratified focal region5.3.2.3 Transformation matrices5.3.2.4 Expression of electric field near focus5.3.3 Numeric simulation results of electric field near focus5.3.3.1 Electric fields at gold/air and glass/air interfaces5.3.3.2 Influence of focus position5.3.3.3 SHG at focus5.3.4 Description of SPR enhanced SHG microscopy6 Conclusion and future workAppendix A Preparation of metal films by thermal evaporationAppendix B Optical constantsB.1 Optical constants of glassAu'>B.2 Optical constant of gold:∈AuCT'>B.3 Optical constant of chromium:∈CTSPR'>Appendix C Extraction of θSPRAppendix D Phosphate solutions of given pH and ionic strengthD.1 Conductivity of phosphate solutions of given pH and ionic strengthAppendix E Matlab codes for the calculation of reflected fieldsAppendix F AbbreviationsPublicationsList of figuresList of tablesBibliography
相关论文文献
- [1].单层膜厚对钙锶铋钛钕薄膜结构及性能的影响[J]. 山东建筑大学学报 2008(01)
- [2].农业单层膜充气温室规模化生产[J]. 中国花卉园艺 2015(06)
- [3].我国建成首栋国产农业单层膜充气温室[J]. 中国花卉园艺 2014(22)
- [4].生物单层膜调控不同形貌方解石单晶生长研究[J]. 河南理工大学学报(自然科学版) 2012(02)
- [5].单层膜充气温室有望在国内的花卉、种苗生产中应用[J]. 中国花卉园艺 2014(02)
- [6].碱性磷酸酶定位方向对DPPC液晶单层膜热力学特性的影响[J]. 陕西师范大学学报(自然科学版) 2017(05)
- [7].牛血清蛋白对二硬脂酰基磷脂酰乙醇胺单层膜结构的影响[J]. 高等学校化学学报 2017(12)
- [8].Ni(111)表面二氧化硫分子单层膜的原子结构[J]. 中国计量大学学报 2019(03)
- [9].化学吸附自组装偶氮苯小分子单层膜及表面浸润性研究[J]. 化学学报 2009(13)
- [10].巯基-金自组装单层膜在生物分析检测领域的技术应用及缺陷与改进方法[J]. 材料导报 2016(23)
- [11].极性自组装单层膜取向铁电液晶器件[J]. 液晶与显示 2009(02)
- [12].十二烷基-β-D-葡萄糖苷单层膜界面性质的分子动力学模拟[J]. 化工学报 2011(11)
- [13].基于紫外光辐照改性自组装单层膜图案化表面的铁酸铋薄膜制备与表征[J]. 硅酸盐学报 2010(02)
- [14].界面单层膜和囊泡双层膜中胆固醇对饱和磷脂膜的影响[J]. 陕西师范大学学报(自然科学版) 2016(03)
- [15].自组装单层膜技术及其在制备功能薄膜领域中的应用[J]. 陶瓷 2009(07)
- [16].自组装单层膜表面的Bi_2Ti_2O_7薄膜制备与表征[J]. 功能材料 2012(11)
- [17].磁控溅射制备的W,WSi_2,Si单层膜和W/Si,WSi_2/Si多层膜应力[J]. 强激光与粒子束 2011(06)
- [18].激光预处理对SiO_2单层膜中缺陷的影响[J]. 中国激光 2010(06)
- [19].温度对TiN/Ti多层膜微观结构和氧化行为的影响[J]. 中国表面工程 2009(05)
- [20].自组装式密堆积的胶体单层膜[J]. 化学研究与应用 2016(07)
- [21].自组装单层膜的研究[J]. 江西师范大学学报(自然科学版) 2009(02)
- [22].自组装单层膜技术制备纳米晶态薄膜的研究[J]. 中国陶瓷工业 2010(05)
- [23].Cu(100)表面N_2O单层膜的原子结构[J]. 科技信息 2012(36)
- [24].表面化学对有限预拉伸衬底上单层膜自组装的影响[J]. 中国科学技术大学学报 2010(06)
- [25].二维纳米金单层膜的构建及其生物电化学应用[J]. 分析化学 2009(09)
- [26].自组装单层膜技术制备纳米晶态薄膜[J]. 材料科学与工程学报 2008(02)
- [27].Cu(100)表面CO分子单层膜的原子结构[J]. 物理学报 2013(18)
- [28].髓鞘碱性蛋白对细胞膜脂质分子DPPC、DPPE单层膜影响机理研究[J]. 中国科学:化学 2014(10)
- [29].C_(90)分子单层膜在Au(111)表面的STM研究[J]. 电子显微学报 2011(Z1)
- [30].Au纳米粒子单层膜表面的等离激元催化反应研究[J]. 光谱学与光谱分析 2016(S1)
标签:芯片论文; 表面等离子体共振论文; 有效介质理论论文; 自组装单层膜论文; 电化学阻抗谱论文; 原子力显微镜论文; 表面二次谐波产生论文;